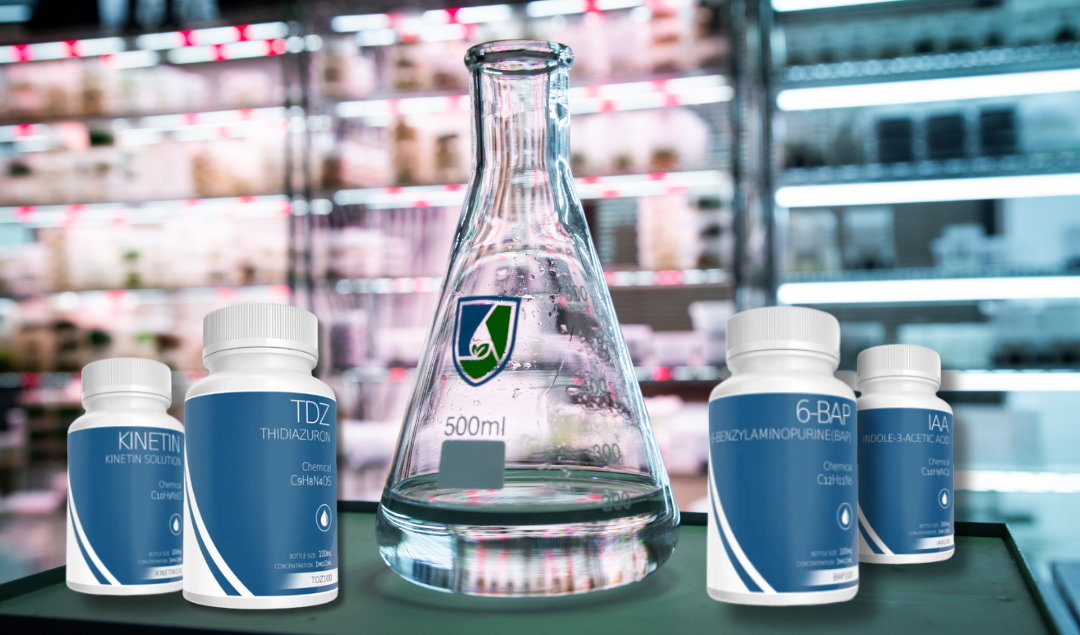
An Advanced Guide On The Role Of Plant Growth Regulators

Introduction
If you're not familiar with the science behind plant hormones or plant growth regulators, mastering tissue culture can be challenging. Knowing how these hormones work and how to balance them properly is essential for a successful experiment.
While we talk so easily about the multiplication and rooting hormones, determining their concentration and role in plant’s growth is very crucial if you are serious about tissue culture.
A million times, we get questions like:
- “Is it okay to use auxin without cytokinin during the multiplication stage?”
- “Should auxin be added during media preparation, or is it better to add it later?”
- “Why do we need to add auxin and cytokinin, and where can we get them?”
- “Do I need to autoclave my hormones?”
Experts might find these questions simple, but for beginners, they can be challenging—and we understand that.
In this article, we will explore the question: 'What role do plant hormones play in tissue culture, and why is understanding them crucial for success?' You'll learn about different plant hormones, their roles in plants, and what kind of hormones are used in tissue culture. We will also explain how to determine the optimum concentration of these hormones and indentify which hormones are autoclavable.
So, read on, Culturists, and deepen your understanding!
What Are Plant Hormones?
Plant hormones, also known as phytohormones or plant growth regulators (PGRs), are naturally occurring organic substances that play a crucial role in regulating plant growth, development, and responses to environmental stimuli, a vital role in tissue culture and plant propagation. Specific plant tissues produce these chemical messengers and transport them to other areas of the plant, where they influence key physiological processes even at low concentrations.
Plant hormones govern a wide range of functions, such as:
- Cell Division and Elongation: Regulating the growth of roots, stems, and shoots.
- Seed Germination: Initiating and controlling the process of seed sprouting.
- Flowering and Fruit Development: Influencing when plants bloom and set fruit.
- Stress Adaptation: Helping plants respond to environmental stress like drought or extreme temperatures.
- Defense Mechanisms: Supporting plant responses to pests and diseases.
- Tropic Responses: Directing plant growth toward or away from environmental stimuli (e.g., light and gravity).

Types of Plant Hormones and Their Functions
There are several types of plant hormones, including auxins, cytokinins, gibberellins, and abscisic acid. Auxins and cytokinins, in particular, are crucial for plant tissue culture, where their balance ensures the proper development of plant organs.
These hormones make it possible to control the growth of plants for agricultural and horticultural purposes, making them essential tools for sustainable crop production and plant propagation.
Auxin
Auxin is a key plant hormone that plays a crucial role in regulating plant growth and development. Discovered by Fritz Went in 1926, the first auxin isolated was indole-3-acetic acid (IAA), which remains the most common form of natural auxin. Young plant tissues, such as leaf primordia, young leaves, and developing seeds, primarily synthesize auxin, which is essential for tissue culture. The plant transports it from the shoots to the roots through specialized pathways in the vascular cambium and phloem, supporting growth and tissue culture applications.
Roles of Auxin in Plants
- Cell Growth and Enlargement: Auxin promotes cell enlargement, particularly in the stem, aiding vertical growth.
- Cell Division: It stimulates cell division in the cambium, contributing to tissue expansion and repair.
- Root Development: Auxin encourages root initiation and branching, which helps plants develop a robust root system.
- Phototropism and Gravitropism: Auxin helps plants respond to light (phototropism) and gravity (gravitropism), guiding their growth toward optimal environmental conditions.
- Apical Dominance: Auxin inhibits the growth of lateral buds (side branches), allowing the main stem to grow taller.
- Delayed Senescence: Auxin delays aging in leaves and other plant tissues, keeping them young for longer and prolonging their functional lifespan.

Cytokinin
Cytokinins are a group of plant hormones that regulate cell division and influence various aspects of plant growth and development. Discovered in the 1950s, cytokinins were first identified when N6-furfuryl aminopurine, later named kinetin, was found to stimulate cell proliferation in Nicotiana tabacum (tobacco) tissue culture.
Researchers isolated this molecule from autoclaved herring sperm DNA extracts. Later, they identified a similar growth-promoting substance, named zeatin, in the immature Zea mays (corn) endosperm. Plants naturally produce cytokinins, mainly in root tips and developing seeds, and transport them to other parts via the xylem.
Roles of Cytokinin in Plants
Cytokinins play several critical roles in plant growth and development:
- Cell Division: Cytokinins, when combined with auxin, induce cell division and trigger callus differentiation into shoots.
- Shoot Formation: They promote shoot initiation and play a vital role in shoot meristem maintenance.
- Leaf Expansion and Delayed Senescence: Cytokinins help in leaf expansion and delay leaf aging, often referred to as leaf senescence.
- Chlorophyll Production: They aid in the accumulation of chlorophyll and convert etioplasts into chloroplasts, improving photosynthesis.
- Root Development: Cytokinins regulate root elongation, lateral root formation, and nodule formation.
- Environmental Response: They modulate plant responses to environmental stimuli, influencing growth patterns like apical dominance.

Gibberellins
Gibberellins (GAs) are a group of plant hormones belonging to the class of tetracyclic diterpenes, essential for regulating growth and development in plants, crucial for tissue culture and plant propagation. Eiichi Kurosawa first discovered gibberellins in 1926 while investigating the 'foolish seedling' disease caused by the fungus Gibberella fujikuroi. Researchers have since recognized their role in promoting plant growth. Over 130 types of gibberellins have been indentified, with GA1, GA3, and GA4+7 being the most active.
Role of Gibberellins in Plants
Gibberellins regulate a wide range of plant physiological processes:
- Stem Elongation: GAs stimulate both cell division and elongation, causing rapid growth in stems.
- Seed Germination: They trigger seed germination, especially in seeds that require cold or light to sprout.
- Enzyme Production: GAs stimulate the production of enzymes like α-amylase in cereal grains, facilitating seedling growth.
- Flowering: Gibberellins promote flowering and fruit development in plants, playing a crucial role in crop yields.
- Male Flower Induction: In dioecious plants, gibberellins help induce male flowers.
The Discovery of Gibberellins
The story of gibberellins began with the “foolish seedling” disease in rice plants in Japan, which caused excessive elongation and poor yield. Kurosawa isolated the active compound from Gibberella fujikuroi, and Yabuta later named it gibberellin in 1935 after crystallizing it. This discovery laid the foundation for our understanding of plant growth hormones and their role in agriculture.

Ethylene
Ethylene is a vital plant hormone that plays a key role in managing growth, development, and aging processes in plants. Most plant tissues naturally produce it, particularly when plants experience stress, such as during injury, ripening, or environmental changes. Ethylene moves through plants via diffusion from its production sites to areas where it exerts its effects.
Role in Plant Growth and Development
Ethylene has a dual nature when it comes to influencing plant growth. At optimal levels, it can encourage growth, but when present in higher concentrations, it can hinder development. For example, low levels of ethylene may promote leaf elongation, while higher levels tend to inhibit this process, especially in certain species. This hormone interacts with other plant hormones to regulate key functions like shoot and root growth, the formation of adventitious roots, and overall plant differentiation.
Leaf Growth and Senescence
Ethylene significantly impacts leaves, influencing both their growth and aging. In some plants, ethylene can promote leaf growth at low concentrations. However, at higher levels or under stress conditions, ethylene can lead to leaf senescence—accelerating the aging process, which eventually results in leaf abscission (falling off). This dynamic balance showcases ethylene’s ability to both promote growth and initiate the aging process, depending on the situation.
Fruit Ripening and Flowering
Ethylene plays a crucial role in these stages, too:
- Flower opening: Ethylene triggers the blooming of some flowers.
- Fruit ripening: Ethylene is a key player in fruit ripening, promoting changes in color, texture, and aroma.
- Leaf and fruit abscission: When leaves or fruits reach maturity or experience stress, ethylene can trigger their detachment from the plant.
Response to Stress and Triple Response
In response to environmental stress, ethylene activates plant defense mechanisms, helping plants adapt and survive. Additionally, it triggers the “triple response” in seedlings, causing a reduction in stem elongation, thickening of the stem, and a shift toward horizontal growth, helping plants navigate obstacles as they grow through the soil.
Abscisic Acid
Abscisic acid (ABA) is a key plant hormone with vital roles in regulating growth, development, and plant responses to stress. One of the most well-studied hormones, ABA influences various processes, from seed dormancy to fruit ripening, and plays a critical role in helping plants cope with both environmental and biological challenges.
Role in Stress Responses
ABA is best known for its ability to help plants manage stress, especially abiotic stresses such as drought, salinity, heat, and cold. When plants experience water shortage, ABA levels increase, leading to the closure of stomata—tiny pores on leaves that control water loss. This response conserves water and prevents dehydration. In saline environments, ABA also helps plants by adjusting ion uptake and balancing osmotic stress. Additionally, ABA plays a crucial role during cold and heat stress by regulating genes that protect plants from damage.
Impact on Growth and Development
In terms of growth, ABA tends to inhibit shoot growth but can promote root development, especially under stress conditions. This dual effect helps the plant focus resources on root growth when water or nutrients are scarce, improving its chances of survival. ABA also regulates seed dormancy, helping seeds withstand unfavorable environmental conditions. By controlling dormancy, ABA ensures seeds only germinate when conditions are optimal for growth.
Fruit Development and Ripening
Beyond stress responses, ABA has a role in fruit development and ripening. During ripening, ABA triggers changes in the fruit, including the breakdown of cell walls, the conversion of starches into sugars, and the production of pigments and aromatic compounds that enhance the fruit’s flavor and appearance. These processes ensure the fruit matures properly and becomes appealing for seed dispersal by animals.
ABA and Interactions with Other Hormones
ABA interacts with other plant hormones, such as ethylene, to balance growth, stress responses, and development. For example, ethylene regulates fruit ripening, while ABA controls the timing and progression of this process, ensuring the fruit reaches full maturity.
In summary, ABA plays an essential role in balancing plant growth, stress management, and development, making it a central player in plant physiology. Its ability to help plants adapt to their environment highlights its importance in agriculture and crop resilience.

Strigolactones
Strigolactones (SLs) are a group of signaling molecules produced by plants with multiple roles, ranging from plant development to symbiotic interactions with soil microbes.
"Researchers discovered them while studying parasitic plants, but they now recognize their critical roles in regulating plant structure, nutrient acquisition, and responses to environmental stress.
Key Functions of Strigolactones
- Plant Hormones: Strigolactones function as endogenous hormones, shaping plant architecture by controlling shoot branching, root morphology, and secondary growth. They regulate the growth or dormancy of axillary buds, influencing overall plant shape. In many plants, they work with other hormones like auxin to suppress excessive branching, a key trait for optimizing resources, especially in agricultural crops.
- Symbiotic Relationships: These compounds also play an essential role in promoting beneficial interactions between plants and microorganisms. Plant roots release strigolactones to attract helpful fungi, called mycorrhizal fungi, which form symbiotic relationships that help the plant absorb essential nutrients like phosphorus and nitrogen from the soil.
- Parasitic Plant Attraction: In some parasitic plants like witchweeds, strigolactones act as a signal that stimulates their seed germination when they are near a suitable host plant. This parasitic function led to the discovery of strigolactones and inspired their name.
Response to Environmental Stress
Strigolactones play a vital role in helping plants adapt to environmental stressors like drought, salinity, and nutrient scarcity. In drought conditions, strigolactones enhance plant resilience by promoting root growth and beneficial symbiosis with microbes like mycorrhizal fungi, aiding in water and nutrient absorption.
Strigolactone signaling also interacts with other hormones, particularly abscisic acid (ABA), to mediate stress responses, such as closing stomata to reduce water loss during drought.
Crosstalk with Other Hormones
Strigolactones don't act in isolation. They interact with several other plant hormones, including ABA, cytokinins, and auxins, to regulate growth and development. For instance, while strigolactones suppress axillary bud growth, cytokinins promote it, creating a balance that shapes the plant's overall structure.
This hormone crosstalk is also crucial for plants to respond flexibly to changes in their environment, such as adjusting growth patterns in response to nutrient availability or water stress.

Brassinosteroids
Brassinosteroids (BRs) are a critical class of plant hormones that influence various aspects of plant growth and development. Researchers first discovered BRs in Brassica pollen and noted their structural similarity to animal steroid hormones. They have since recognized BRs for their role in regulating plant responses to both internal growth signals and environmental stresses."
Biosynthesis and Signaling:
BRs are synthesized in various plant tissues, particularly in the stems and roots, through a multi-step biosynthesis pathway.
The biosynthesis pathway involves multiple steps, including the conversion of cholesterol to brassinosteroids. BRs bind to specific receptors on the cell surface, triggering a signaling cascade that ultimately leads to changes in gene expression and plant growth.
Key Functions of Brassinosteroids:
- Cell Elongation and Division: Promote overall plant growth by regulating cell expansion and proliferation.
- Root and Vascular Development: Support root growth, improve nutrient uptake, and enhance vascular differentiation.
- Seed Germination: Stimulate seed germination and early plant development.
- Reproductive Processes: Play a role in floral transition, anther development, and pollen maturation.
- Stress Response: Help plants respond to environmental challenges such as drought, salinity, heat, cold, and nutrient deficiency.
- Immunity and Defense: Regulate the balance between growth and defense, optimizing resource allocation for survival.
In agriculture, BR analogs have demonstrated the potential to boost crop yields and stress resilience, making them valuable for improving plant productivity under adverse conditions. Understanding their mechanisms can aid in developing better breeding strategies and agricultural practices.

Salicylic Acid
Salicylic acid (SA) is a critical phytohormone with key roles in plant immunity, growth, and development. Known for its function in triggering plant defense mechanisms, SA is synthesized via two primary pathways: the isochorismate (IC) pathway, predominant in Arabidopsis, and the phenylalanine ammonia-lyase (PAL) pathway, which is dominant in rice. SA plays a dual role in regulating both plant growth and immune responses, making it an essential component of plant health.
Key Functions of Salicylic Acid:
- Immunity and Defense: SA is central to a plant’s immune system. It activates the production of pathogenesis-related proteins, which help plants resist pathogens. SA also triggers systemic acquired resistance (SAR), where a pathogen attack on older leaves induces immune responses in younger leaves, providing whole-plant protection against future attacks.
- Growth Regulation: SA influences plant growth by regulating cell division and expansion. Its effects vary depending on its concentration. High levels of SA often lead to stunted growth and dwarf phenotypes, as seen in certain Arabidopsis mutants, whereas low levels of SA can promote larger biomass production. For example, SA-deficient plants tend to show increased leaf and stem growth compared to wild-type plants.
- Thermogenesis: In plants like the voodoo lily (Sauromatum guttatum) and Arum, SA contributes to thermogenesis, a process where plants generate heat. This helps attract pollinators in certain species by raising the temperature of flowers, a unique biological role of SA.
- Concentration-Dependent Growth Effects: The impact of exogenous SA on plant growth is highly dependent on its concentration and plant species. Lower concentrations of SA generally promote growth, such as increasing leaf size and promoting root development. In contrast, higher concentrations of SA can inhibit growth, reducing leaf and root size in species like barley and Arabidopsis. For example, in wheat, low SA levels stimulate growth, while higher levels have a suppressive effect.
SA's ability to balance growth and defense responses makes it an essential hormone for maintaining plant health and resilience.

Jasmonic Acid
Jasmonic acid (JA) is a prominent phytohormone that influences plant growth, development, and defense mechanisms. As part of the jasmonates family, JA helps plants respond to both biotic (like pathogens and herbivores) and abiotic stresses (such as heat, drought, and salinity). Its roles extend to various developmental stages like seed germination and leaf senescence, making it a critical regulator in plant biology.
Biosynthesis of Jasmonic Acid
The octadecanoid pathway produces JA, starting from α-linolenic acid in chloroplast membranes. Several enzymes, such as lipoxygenase (LOX) and allene oxide synthase (AOS), help convert these fatty acids into 12-oxophytodienoic acid (OPDA), a precursor of JA. The pathway ends in the peroxisomes, where the plant processes OPDA into JA. This tightly controlled biosynthesis often activates in response to environmental stress, allowing the plant to quickly respond to external threats by producing JA.
Functions of Jasmonic Acid
JA’s functions in plants are twofold: it acts as a key player in growth regulation and as a defense mediator. In development, it regulates processes such as root formation, flowering, and senescence. JA’s most notable role, however, is in defense, where it helps the plant build resistance against herbivores and necrotrophic pathogens. In response to abiotic stresses like drought or high salinity, JA modulates antioxidant enzyme activities, protecting cells from oxidative stress and improving plant tolerance.
Role in Agriculture and Tissue Culture
In agriculture, JA’s ability to enhance plant resistance to various stresses has made it invaluable for improving crop resilience. Applying exogenous JA boosts the plant’s natural defense mechanisms, resulting in higher stress tolerance and improved yields under challenging environmental conditions. Tissue culture utilizes JA to trigger specific responses like root formation and secondary metabolite production, further showcasing its value in controlled plant development and genetic engineering efforts.

What Type of Hormones Are Used in Tissue Culture, and If They Are Autoclavable?
Auxin
Plant tissue culture (PTC) relies heavily on auxin for several processes, from promoting root growth to ensuring proper organ differentiation. Auxins are essential in creating the right balance of hormones required for the growth of plant tissues in a controlled environment. Some common types of auxins used in tissue culture include:
Indole-3-Acetic Acid (IAA)
Tissue culture often uses IAA, the primary natural auxin, to promote cell elongation and root initiation. Although effective, it degrades quickly and requires sterilization before use to maintain a sterile environment.
Indole-3-Butyric Acid (IBA)
IBA is a synthetic auxin often used in plant tissue culture for root development. It is more stable than IAA, making it suitable for longer-term projects. Like IAA, it needs to be sterilized before application.
Naphthaleneacetic Acid (NAA)
NAA is another synthetic auxin, widely used in PTC to promote both root and shoot development. It has a longer-lasting effect compared to natural auxins and is ideal for a variety of plant species. NAA is also sterilized to ensure that no contaminants affect the culture.
2,4-Dichlorophenoxyacetic Acid (2,4-D)
2,4-D is commonly used in tissue culture, particularly for inducing callus formation and somatic embryogenesis. This synthetic auxin is highly potent and must be carefully sterilized and handled to maintain precise hormone levels.
How Auxins Are Used in Tissue Culture
In tissue culture, the balance between auxins and cytokinins determines the type of growth—auxin promotes root growth, while cytokinin encourages shoot development. Auxins are sterilized before use to ensure they don’t introduce contaminants into the culture, preserving the controlled environment necessary for successful plant propagation.
In conclusion, auxins play an indispensable role in plant tissue culture, helping to propagate healthier and genetically improved plants. Whether natural or synthetic, the precise use of these hormones is essential for the efficient production of high-quality plants.

Cytokinins in Tissue Culture
In plant tissue culture (PTC), cytokinins are crucial for inducing cell division and promoting shoot development. Their use is often combined with auxins to balance cell growth and differentiation. Cytokinins help in producing multiple shoots, enabling the efficient propagation of plants with desired genetic traits.
Types of Cytokinin Used in Tissue Culture
-
Kinetin
Kinetin is one of the earliest cytokinins discovered and is widely used in tissue culture to stimulate cell division and shoot initiation. It’s particularly effective in promoting shoot proliferation and inducing callus differentiation. Sterilization of kinetin typically occurs through autoclaving or filter sterilization before its use in culture media. -
Zeatin
Zeatin, another natural cytokinin, promotes shoot initiation and enhances overall plant growth It plays a pivotal role in regulating cell division and delaying senescence in tissue culture. Like kinetin, zeatin is sterilized using filtration or autoclaving to maintain a sterile environment during tissue culture procedures. -
Benzylaminopurine (BAP)
BAP, a synthetic cytokinin, is widely used in tissue culture for its ability to stimulate multiple shoot formation and enhance shoot elongation. Effective at low concentrations, it is often preferred for commercial plant propagation. Sterilizing BAP before adding it to culture media ensures contamination-free tissue culture operations.
How Are Cytokinins Used in Tissue Culture?
Cytokinins are incorporated into plant tissue culture media to promote specific growth responses, such as shoot multiplication or callus formation. The concentration of cytokinins in the medium is carefully controlled to balance their effects with other hormones like auxins, depending on the desired outcome (e.g., root formation or shoot proliferation). Techniques like autoclaving or filter sterilization sterilize cytokinins to prevent contamination in the highly sensitive tissue culture environment.
By leveraging cytokinins in tissue culture, researchers and horticulturists can efficiently propagate plants with desired traits, enabling the mass production of disease-free, genetically improved plants for agricultural and horticultural use.

Gibberellins in Tissue Culture
Gibberellins, particularly GA3, play a specific role in plant tissue culture. Although around 126 gibberellins are known, only a few are used in tissue culture due to their selective effects on plant development.
GA3 in Tissue Culture
- Inducing Callus Growth: GA3 is essential for promoting callus growth in many tissue culture operations. It aids in forming undifferentiated plant cells, which can develop into various plant tissues.
- Adventitious Root Formation: Gibberellins stimulate the growth of adventitious roots, supporting the regeneration process in tissue culture.
Avoiding Gibberellins in Organ Development
While gibberellins are helpful in certain processes, they can inhibit others, such as organ formation (roots and shoots) and somatic embryogenesis. This makes their use in tissue culture highly specialized, depending on the plant species and the desired outcome.
Types of Gibberellins Used in Tissue Culture
- GA3 (Gibberellic Acid 3):
- Usage: Primarily used to induce plantlet formation and promote seed germination.
- Sterilization: Often sterilized before use in tissue culture to avoid contamination.
- GA4+7:
- Usage: This combination is applied to stimulate shoot growth and promote flowering.
- Sterilization: Like GA3, it is sterilized to ensure a contaminant-free culture medium.

Your One-Stop Shop For All Things Plant Tissue Culture
Revolutionizing the industry, Plant Cell Technology offers a range of exceptional products and services to optimize your workflow. Our store features essential items, including all essential plant growth hormones, MS media, agar, gellan gum, Plant Preservative Mixture™ (PPM™), culture vessels, and Biocoupler™, as well as additional supplies like masks.
Beyond providing materials, our consultation services aim to deliver instant solutions to your tissue culture challenges. If you're seeking expertise to enhance your skills, our tailored tissue culture master classes provide comprehensive learning directly from the experts.
These courses encompass a wide range of topics, covering fundamental concepts and hands-on experiences, such as explant and media preparation, multiplication, rooting, and acclimation of plants.
Additionally, the curriculum includes advanced techniques like shoot apical meristem and synthetic seed production, ensuring a comprehensive learning experience to make you proficient in the industry. Taught by tissue culture experts with 10-30 years of experience, our masterclasses not only educate on tissue culture concepts but also share personal success and failure stories, providing valuable insights for your journey.
Ready to advance your tissue culture skills? Waste no time and enroll in your preferred tissue culture master class today to enhance your expertise in the field!
Blog Categories
View by Level
Popular Blogs

Common Mistakes in Plant Tissue Culture and How to Fix Them
Introduction Tissue culture offers a fascinating way to regenerate plants, unlocking immense potential for growth and sustainability However, even the...
Read More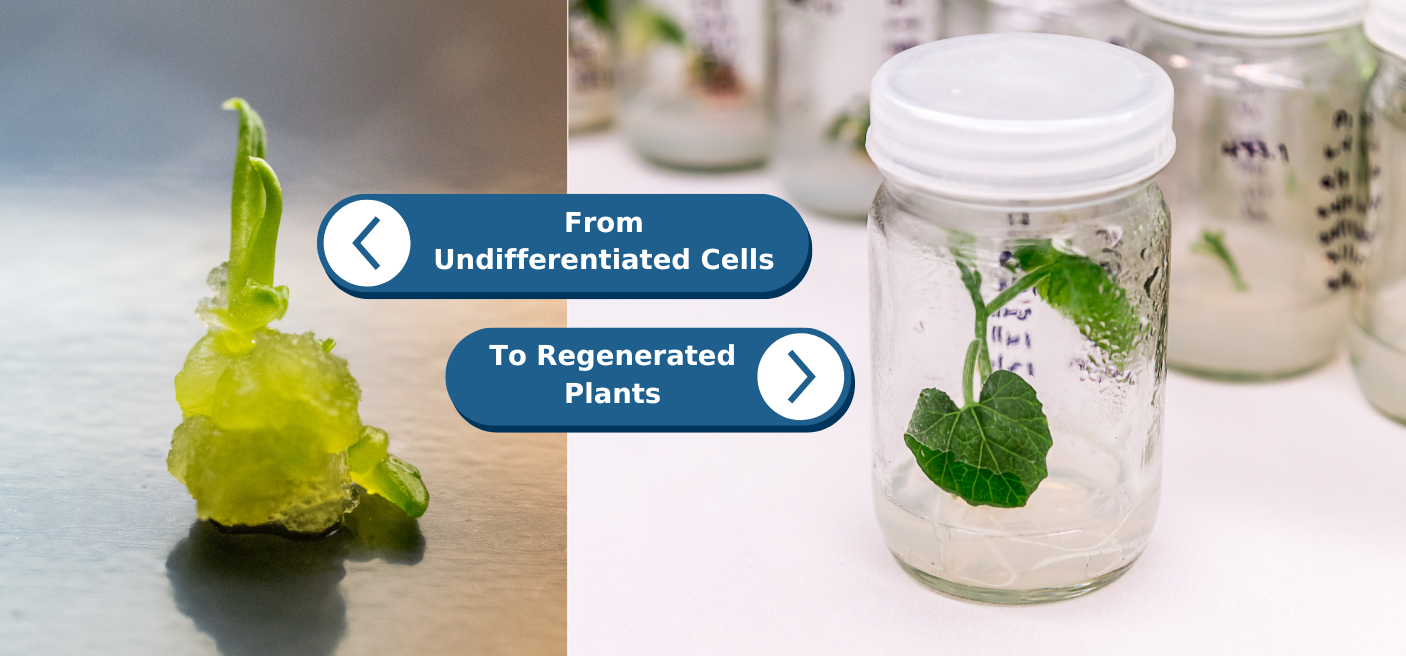
Callus Culture: From Undifferentiated Cells to Regenerated Plants
Introduction Plant tissue culture holds immense potential in horticulture and agriculture, offering transformative applications. While many recognize its benefits, fewer...
Read More1 comment
I’m wanting to build my own PGR’s for field corn production
Anyone willing to assist?
Join the conversation
Your email address will not be published. Required fields are marked